Scientific Related Articles
Patent Pending Inventors – Dr. Alonso Moreno, Ian Harvey, Brian Baker and Howard Leonhardt
- Biological pacemaker growing chamber on tip of catheter
- Sino-atrial node cell implanted provide bioelectrical signal
- Molecular and genetic biological solutions to stabilize implanted pacemaker cells
- Bioelectric signals to regulate biological pacing
Related Articles
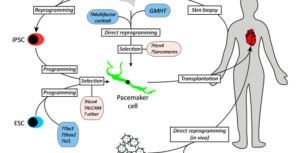
Nov 16, 2017 – Biological pacemakers, generated by somatic gene transfer, cell fusion, or cell transplantation, provide an alternative to electronic devices.
Natl Med J India. 2017 Nov-Dec;30(6):324-326. doi: 10.4103/0970-258X.2
Sep 6, 2011 – The biological pacemaker, a tissue that spontaneously or via engineering confers pacemaker properties to regions of the heart, is an exciting …
Jul 16, 2014 – Biological pacemaker created by minimally invasive somatic reprogramming in pigs with complete heart block. Yu-Feng Hu,,; James Frederick …
Jul 9, 2019 – (B) Horizontal and vertical planes of the perfusion chamber with electrical stimulation. The cardiac construct (scaffold + cells; pink) is held in place by two gaskets (blue). … The bioreactor supports the culture of up to four cardiac constructs …. Human cardiac macrotissues produced a bioelectrical signal that .
The shear stresses exerting on the bioreactor chamber were calculated by … Tissue engineering is a multidisciplinary field that aims to construct biological tissues such as … Bioreactors and scaffolds including hydrogels play critical roles in tissue …. When matrixproduction by the chondrons is visualized with an alcian blue .
Mar 16, 2018 – Our simplified electro-bioreactor is customized for applying DC … This device is applicable to various types of biological and biomedical studies that investigate the effects of bioelectrical stimulation and its applications. … dishes in CO2 incubators or in 3-D scaffolds in bioreactors that more …. Matrix Biol.
Dec 4, 2015 – Shear stiffness during LV contraction closely matches chamber pressure …… Stem cell-based biological pacemakers from proof of principle to …
Jun 20, 2015 – Keywords: Scaffold, Pore size, Tissue regeneration, Stem cells ….. Porous membrane in dual-modified Boyden chamber, 3, hMSC, No, Towards ….. The pore sizes for the biological pacemaker should allow some contact of .
Jan 21, 2019 – Functional biological pacemaker generation by T-Box18 protein expression via stem cell and viral delivery approaches in a murine model of …
Natl Med J India. 2017 Nov-Dec;30(6):324-326. doi: 10.4103/0970-258X.239072. Biological pacemakers: Concepts and techniques. Ambesh P(1), Kapoor A(2).
Claims (31)
What is claimed is:
1. A method for providing for pacing of a human heart of a patient comprising:
a. isolating a plurality of viable sino-atrial node cells from a human heart; and,
b. implanting said plurality of viable sino-atrial node cells within said human heart, whereby said implanted viable sino-atrial node cells pace said human heart.
2. A method according to claim 1 wherein said implanting step further comprises implanting said plurality of viable sino-atrial node cells within a ventricle of said human heart.
3. A method according to claim 2 wherein said implanting step further comprises implanting said plurality of viable sino-atrial node cells within the right ventricle of said human heart.
4. A method according to claim 3 wherein said implanting step further comprises implanting said plurality of viable sino-atrial node cells within the apex of said right ventricle of said human heart.
5. A method according to claim 4 wherein said plurality of viable sino-atrial node cells are from said patient.
6. A method according to claim 5 further comprising, after said isolating step and before said implanting step, removing said plurality of viable sino-atrial node cells from said patient.
7. A method according to claim 6 further comprising, after said removing step and before said implanting step, culturing said plurality of viable sino-atrial node cells.
8. A process for providing a biological pacemaker implant for the human heart which comprises the steps of:
a. identifying S-A node cells in the right atrium of the heart;
b. removing a quantity of said S-A node cells from the wall of the right atrium;
c. culturing said quantity of S-A node cells to grow a quantity of said S-A node cell to produce a critical mass of said S-A node cells sufficient to generate a depolarization wave in myocardial tissue; and,
d. implanting said critical mass of S-A node cells in the right ventricle of the heart to provide a depolarization wave of sufficient magnitude to stimulate contraction of the myocardial tissue of the ventricles of the heart to initiate and sustain substantially natural heartbeat.
9. A process in accordance with claim 8 in which the quantity of S-A node cells is removed from and implanted in the same heart.
10. A process in accordance with claim 8 in which culturing of said S-A node cells is carried out in-vitro and in a culture medium compatible with culture of myocardial tissue.
11. A process in accordance with claim 8 in which said quantity of S-A node cells is temporarily transplanted in a temporary site of the patient’s body and in which said culturing takes place at said temporary site for a sufficient period of time to grow said critical mass of S-A node cells after which said critical mass of S-A node cells are removed from said temporary site for implanting in said right ventricle.
12. A process in accordance with claim 8 in which said critical mass of S-A node cells is implanted in the wall of the lower two thirds of the right ventricle.
13. A process in accordance with claim 8 in which said critical mass of S-A node cells is implanted at the apex of the myocardial tissues of the right ventricle of the heart.
14. A process in accordance with claim 8 which further includes the step of destroying the S-A node cells remaining in the right atrium to prevent generation of a depolarization wave out of synchronization with the depolarization wave generated by said implanted mass of S-A node cells.
15. A process in accordance with claim 14 in which said S-A node cells remained in said right atrium are cryogenically destroyed.
16. A process in accordance with claim 14 in which said S-A node cells remaining in said right atrium are destroyed through electrical ablation.
17. A process for providing a biological pacemaker implant for the human heart which comprises the steps of:
a. inserting a mapping catheter into the right atrium of the heart;
b. mapping the site of the S-A node cells of the right atrium to determine the boundaries of the S-A node site and to identify the location of healthy S-A node cells;
c. removing a quantity of said healthy S-A node cells from the site of said S-A node cells in the right atrium;
d. culturing said quantity of S-A node cells to grow a quantity of said S-A node cell to produce a critical mass of said S-A node cells sufficient to generate a depolarization wave in myocardial tissue; and,
e. implanting said critical mass of S-A node cells in the right ventricle of the heart to provide a depolarization wave of sufficient magnitude to stimulate contraction of the myocardial tissue of the ventricles of the heart to initiate and sustain substantially natural heartbeat.
18. A process in accordance with claim 17 in which the quantity of S-A node cells is removed from and implanted in the same heart.
19. A process in accordance with claim 17 which further includes the step of recording the boundaries of the site of the S-A node cells in the right atrium.
20. A process in accordance with claim 19 which further includes the steps of locating the site of the S-A node cells in said right atrium from said mapping of said sites and destroying the S-A node cells remaining in the right atrium to prevent generation of a depolarization wave in the right atrium.
21. A process in accordance with claim 17 in which said critical mass of S-A node cells is implanted at the apex of the myocardial tissues of the right ventricle of the heart.
22. A process in accordance with claim 17 in which said critical mass of S-A node cells is implanted in the wall of the lower two thirds of the right ventricle of the heart.
23. A process for providing a biological pacemaker implant for the human heart which comprises the steps of:
a. identifying S-A node cells int he right atrium of the heart;
b. removing a quantity of said S-A node cells from the wall of the heart;
c. implanting a pacemaker electrode in the right ventricle of the heart;
d. periodically inducing a depolarization wave in the myocardial tissue of the right ventricle through said electrode to promote a regular heartbeat function in the heart;
e. culturing said quantity of S-A node cells to grow a quantity of said S-A node cell to produce a critical mass of said S-A node cells sufficient to generate a depolarization wave in myocardial tissue;
f. implanting said critical mass of S-A node cells in the right ventricle of the heart to provide a depolarization wave of sufficient magnitude to stimulate contraction of the myocardial tissue of the ventricles of the heart to initiate and sustain substantially natural heartbeat of the heart; and,
g. removing said pacemaker electrode from the right ventricle of the heart.
24. A process in accordance with claim 23 in which the quantity of S-A node cells is removed from and implanted in the same heart.
25. A process in accordance with claim 23 in which culturing of said S-A node cells is carried out in-vitro and in a culture medium compatible with culture of myocardial tissue.
26. A process in accordance with claim 23 in which said quantity of S-A node cells is temporarily transplanted in a temporary site of the patient’s body and in which said culturing takes place at said temporary site for a sufficient period of time to grow said critical mass of S-A node cells after which said critical mass of S-A node cells are removed from said temporary site for implanting in said right ventricle.
27. A process in accordance with claim 23 in which said critical mass of S-A node cells is implanted in the wall of the lower two thirds of the right ventricle.
28. A process in accordance with claim 23 in which said critical mass of S-A node cells is implanted at the apex of the myocardial tissues of the right ventricle of the heart.
29. A process in accordance with claim 23 which further includes the step of destroying the S-A node cells remaining in the right atrium to prevent generation of a depolarization wave out of synchronization with the depolarization wave generated by said implanted mass of S-A node cells.
30. A process in accordance with claim 29 in which said S-A node cells remaining in said right atrium are cryogenically destroyed.
31. A process in accordance with claim 29 in which said S-A node cells remaining in said right atrium are destroyed through electrical ablation.
DESCRIPTION
This is a continuation of application Ser. No. 07/319,094, filed Mar. 6, 1989, now abandoned.
BACKGROUND OF THE INVENTION
A major cause of death and poor health in significant segments of the population in the United States and in many other areas of the world involve disease and insufficient function of the heart. The vitality of all tissues in the body depends upon a continual flow of blood at an adequate rate to permit efficient and satisfactory function of the organs. The heart is required to function at a relatively high level. The heart typically pumps 75 gallons of blood per hour when the body is at rest and is required to function at even higher rates during moderate or heavy levels of exertion and activity.
Interruption or interference with the continuous and efficient function of the heart can occur for a variety of reasons. The arteries of the heart may become diseased and obstructed with the result that the heart will either develop insufficient blood flow or blood flow will become terminated. This arteriosclerosis is the well-known coronary artery disease that is a leading killer of some segments of the population, especially men.
Diseased coronary arteries often provide restricted circulation and diminished blood flow with the result that the heart is unable to carry out its normal function as it is gradually starved for blood. The result is that the heart fails to contract as forcefully as necessary with the result that the entire body suffers from insufficient blood flow.
When one of the coronary arteries becomes plugged by a blood clot, that particular area of the heart served by the plugged coronary artery will be cut off from an adequate supply of blood and, if circulation is not immediately resumed, the muscle tissue of that particular area of the heart will become impaired or die.
Insufficient or terminated blood flow in certain areas of the heart can also have deleterious effect on other functions of the heart, including the conduction system of the heart. The conduction system of the heart is a group of structures within the heart that determine heart rate in response to influences from the nervous system as well as the chemical information carried to the heart from other organs of the body. The conduction system provides stimulating impulses to all parts of the myocardium in a coordinated fashion. The coordination of the impulses is important to ensure that the different sections of the heart act in coordination to pump blood throughout the body. Coordinated function of the heart contraction ensure delivery of an adequate supply of blood to the various organs as demands on the organs vary. The stimulations necessary for proper excitation of the myocardium need to be coordinated to ensure the heart contracts effectively to make the heart an effective fluid pump.
The conduction system in the heart depends upon a regular generation of a depolarization wave of adequate magnitude to cause the myocardium to contract in an orderly fashion to force blood through the body’s veins and arteries. The proper function of the heart is dependent upon the ability of the heart to generate or start a depolarization wave at a particular location in the heart in order to ensure a proper contraction. This depolarization wave must be generated in a place, at a location and with a frequency which is responsive to the needs of the heart as well as the other functions of the body.
The depolarization wave of the heart is generated as a result of some unique characteristics of myocardial tissue. Depolarization occurs as cell tissue, either muscle or nerve cells, is stimulated. The stimulus is then transmitted to the next cell in a process which is called depolarization. Through this process, a depolarization wave can be generated in a mass of muscle tissue with the result that the muscle tissue responds to the stimulus. This response results in the familiar muscle function or heart beat of the heart.
It has been learned that the generation of this depolarization stimulus is a characteristic of the behavior of the cell membrane of individual cells of living tissue. Living cells selectively permit the passage of various substances such as nutrients, oxygen, waste products through the cell membrane. These substances move freely through the cell membrane in order to ensure the adequate nutrition of the cell and maintain the life function of the cell. While there is a free movement of substances through the cell membrane, the movement is by no means unrestricted. Certain essential substances are blocked by the cell membrane so that certain essential substances are not permitted to move outside of the cell.
Other substances are not permitted to move from outside of the cell to the interior of the cell with the result that there is a substantial selectivity which occurs at the cell membrane preventing movement of selected elements through or across the cell membrane depending upon the nature of the substance. As an example, this selective permeability at the cell membrane
Could the Biological Pacemaker Replace the Mechanical Version?
Share on facebookShare on twitterShare on emailShare on printMore Sharing Services0
Posted in Cardiovascular by Brian Buntz on July 17, 2014
The pacemaker of the future could be biological, according to scientists at Cedars-Sinai Heart Institute. The researchers have developed a method of converting cardiac muscle cells into pacemaker cells, and have shown that it is effective in a limited animal trial. Hypothetically, the therapy could be used to treat patients who are poor candidates for traditional pacemaker devices.
In a trial, which was described in the Science Translational Medicine journal, the researchers explain how they were able to reestablish normal heart rates in pigs with a condition known as heart block. Often treated with mechanical pacemakers in humans, heart block causes an extremely slow heart rate.
To treat the condition in pigs, the scientists injected a gene known as Tbx18 that triggers the growth of pacemaker cells during fetal development. Because the gene is not normally active after birth, the researchers used a virus as a vector to introduce the gene into the heart, where it coaxed a small area of the heart—roughly the size of a peppercorn—to form a new sinoatrial node. That development led to the formation of a new functional biological pacemaker in an area of the heart not usually responsible for initiating the pulse.
Refresh your medical device industry knowledge at MEDevice San Diego, September 10–11, 2014.
The researchers were able to inject the gene, packaged inside of an adenovirus vector, into the animals’ right ventricle using a catheter rather than open-heart surgery. Although adenoviruses are linked to a number of infections—ranging from tonsillitis to ear infections, the viral vector used here was engineered to be harmless, incapable of spreading to outside of the heart target.
One of the limitations of the research so far is its duration. In the trial on pigs, the treatment proved to be effective for two weeks. After that time frame, the heart rates of the pigs treated with the gene therapy began to decrease.
Still, the scientists think that as the research advances, the duration could improve. “We don’t have any reason to believe… that the two weeks is somehow a magic cap,” said researcher Eduardo Marbán, MD, director of the Cedars-Sinai Heart Institute in a statement. As the research evolves, the scientists could develop a more durable biological solution. “These results show us that with more research, we might be able to develop a long-lasting biological treatment for patients.”
In any event though, the treatment method could ultimately provide at least a short-term treatment for heart patients in need of pacemaker treatments that are poor candidates for traditional devices.
It also has the potential to treat infants with congenital heart block—a rare but often fatal disorder. “Babies still in the womb cannot have a [mechanical] pacemaker, but we hope to work with fetal medicine specialists to create a life-saving catheter-based treatment for infants diagnosed with congenital heart block,” explained Eugenio Cingolani, MD, the director of the Heart Institute’s Cardiogenetics-Familial Arrhythmia Clinic in a statement. “It is possible that one day, we might be able to save lives by replacing hardware with an injection of genes,” he said.
The scientists at Cedars-Sinai Heart Institute plan on performing human trials of the gene-therapy procedure in three years or so.
The researchers stressed that the gene-therapy pacemaker treatment was promising in that could help people with heart rhythm disorders who also had side effects such as infections of leads stemming from traditional pacemakers.
Already though, device firms such as Medtronic and St. Jude (with its Nanostim division) are working on commercializing small, leadless catheter-implanted pacemakers.
Pacemaker Researchers Swap Batteries for Biology – Click Here
Patent US5103821 – Method of providing a biological – Click Here
BioPace – T he world’s first biological pacemaker made of living cells – http://leonhardtvent.wpengine.com/biopace.php
Elevator Pitch: The first heart pacemaker made entirely of your own living cells just like natural pacemakers. No batteries to go dead. No steel cans. No steel leads. Set to obsolete the multi-billion steel can steel lead pacemaker industry.
Problem: Steel can pacemakers are costly and suffer risks of battery depletion, toxic reactions, infections and lead breaks.
Solution: BioPace builds a pacemaker from your own cells that will last forever and is far less costly. Restores normal pacing. Works exactly how natural pacemakers work.
Stage of Development: Completed dog study. Preparing for historic landmark first-in-man case.
Forecasted Sales Year 1 = $300 million, Year 2 $800 million, Year 3 $2 billion, Year 4 $4 billion, Year 5 $5 billion
Management Team: Howard Leonhardt CEO, Wendell King COO, Dr. Eric Duckers CSO, Dr. Sergio Pinski CMO.
Lead Scientists: Dr. Eric Duckers Utrecht University, Dr. Sergio Pinski Cleveland Clinic Florida
Strategy: Launch OUS first in 2016 and then in U.S. in 2018.
Business Model: Sell product to electrophysiologists and cardiologists. OUS via distributors. U.S. via 55 independent sales reps part of www.heartscore.co ;
Patents: 2 pioneering patents issued. 2 additional patents in process.
Target Exit and Likely Buyers List: $2 billion to Medtronic, St. Jude, Boston Scientific, Johnson & Johnson, Abbott Labs, Terumo, Thoratec, Heartware, Biotronik, Sorin, Amgen, Terumo, Novartis or Edwards in 2018.
Near Term Milestones:
- Complete pre-clincial studies.
- Complete historic landmark first-in-man implantation
- Current valuation = $3 million. Target valuation 2018 = $2 billion.